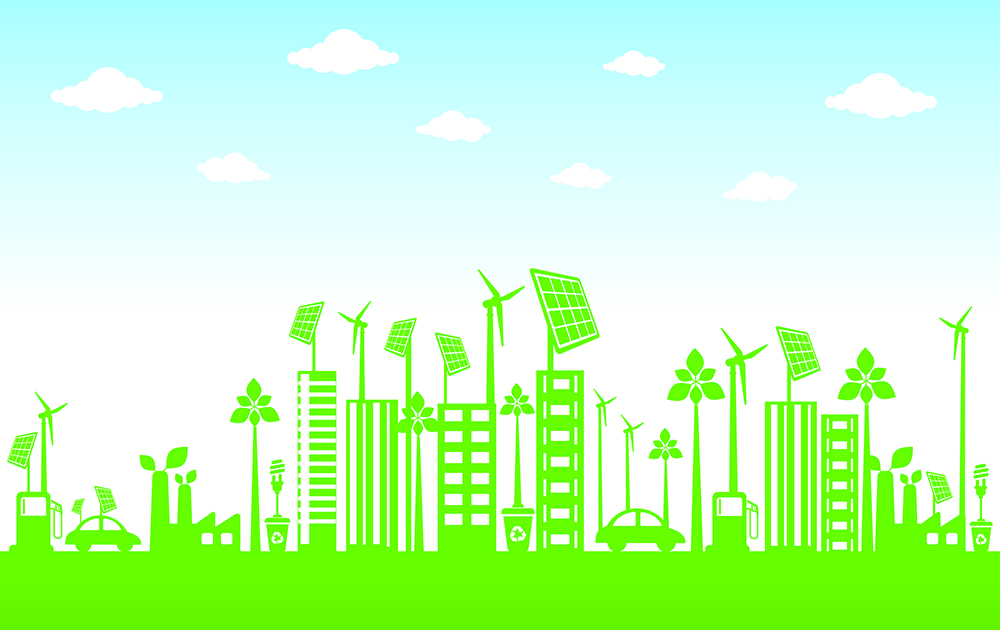
At 11.9%, road transport is the world’s single biggest greenhouse gas emitter (namely carbon dioxide equivalents) and its decarbonisation is imperative in combatting climate change. The transformation will require systemic changes which will impact many national and local government departments, as well as energy providers and a plethora of small businesses.
For more than a century, the internal combustion engine (ICE) has almost exclusively powered road transport. Passenger transport (predominantly private cars) contributes 60% of the emissions released - the remainder comes from freight.
ICE vehicles could deliver zero tailpipe emissions by running on hydrogen but the overall efficiency would be much lower than pure electric contenders: hydrogen fuel cell electric vehicles (HFEVs) and battery-powered vehicles (BPVs).
HFEVs produce zero carbon at the tailpipe but otherwise fit comfortably in the petrol/diesel footprint in terms of the refuelling network requirements, time taken to refuel, distance between refuelling and weight, while BPVs are the complete opposite. BPVs’ batteries are big, the vehicles are heavier than their ICE/HFEV equivalent and charge times are longer although they can be recharged at home, a depot and suitably equipped service stations. BPVs are also more efficient than their ICE and HFEV counterparts.
Both BPVs and HFEVs are suitable for non-intensive uses including private cars (the biggest CO2 contributor) and light vans, and both use electric traction motors. The difference is in the energy type and storage: BPVs use lithium-based batteries to store electrical energy while HFEVs carry compressed hydrogen (H2) which is recombined with oxygen from the atmosphere in the fuel cells to produce electricity (and water).
In energy-density terms, lithium-ion batteries currently store around 0.250kWh/kg while diesel contains 12.5kWh/kg of energy and petrol 12.8kWh/kg. Every kilogram of H2 contains more than 33kWh.
BPVs’ battery packs are large and heavy, limiting the range of small battery-powered cars (BPCs) like the Mini which can cover 233km (145 miles) on a charge, while Mercedes’ pure electric SUV - the EQS - can cover an ICE-equalling 728km (453 miles).
With 6.3kg of H2 on board, the range of Hyundai’s Nexo HFEV is around 640km (400 miles). Nonetheless, BPVs are the most efficient (see below) although the battery’s additional weight and space requirements, restricted range and longer ‘refuelling’ time mean hydrogen fuel cells are more suitable for high-use and commercial vehicles.
For governments and departments of transportation, the questions are, what can they do to guide and facilitate the decarbonisation of transport and what will be the wider consequences of the move away from fossil fuels?
Fuel for thought
Hydrogen can be produced in many ways including electrolysis – with nine litres of water making 1kg of H2 that would power Hyundai’s Nexo car for 100km (62 miles).
Refuelling with compressed H2 takes about the same time as with petrol and diesel while, even using a fast three-phase DC charger, it still takes half an hour to boost the battery of most electric cars from 10% to 80% (even longer for commercial vehicles).
As petrol and diesel are phased out, fuel stations will find themselves at the sharp end of decarbonisation and could replace each fuel pump with an H2 refuelling point with the compressed hydrogen delivered via road tanker. However, in terms of efficiency and road safety, it would (space permitting) be better to produce and store the H2 on site. This would require upgrading the electrical supply and a possible revision of national and local planning and safety regulations.
To maintain ICE vehicle levels of throughput with BPCs, each petrol pump would have to be replaced by at least five fast chargers – a move that would massively increase the site’s power consumption. However, with a suitable power supply, existing service stations could provide both BPV and H2 refuelling facilities. Even with a favourable regulatory framework, this will be financially and technically challenging for many businesses.
Even after such upgrades, service stations must expect a fall in business as most BPC recharging will be done at the user’s home – at least those with off-street parking. To ease this problem many local authorities are installing chargers at the roadside and in car parks and they could also consider regulatory changes allowing embedded gullies in pavements outside dwellings without off-street parking to carry power leads to BPVs.
‘Range anxiety’ hampers EV sales and deters BPV owners from making longer journeys – a problem not addressed by the slow AC recharging points authorities are installing, which need many hours to recharge vehicles.
To increase BPV acceptability, especially with smaller vehicles, authorities need to implement policies that will foster a readily-available network of fast DC chargers that enable BPV drivers to reach their destination in a reasonable time. These three-phase devices draw a lot of power and a network of them will have a significant impact on electricity producers and distribution systems.
The European Union’s Hydrogen Roadmap identifies hydrogen as ‘the most promising decarbonisation option for trucks, buses, ships, trains, large cars and commercial vehicles’. So even with a high-speed BPV charger network, there will still be a need for an H2 refuelling network.
Due to their cost and rigorous regulatory requirements, H2 refuelling sites are unlikely to spring up organically.
In 2013, the UK government estimated 65 H2 refuelling stations would be operating by 2020 but by 2022 there were just 14 with only an estimated 300 hydrogen fuel cell vehicles (mainly cars and buses) on the roads. Recently, Shell UK closed the three H2 refuelling stations it operated (citing lack of demand) and is refocusing its hydrogen refuelling business on trucks and buses. It will also install 100,000 rapid DC chargers by 2030.
Research shows HFEV drivers love their vehicles but bemoan the lack of publicly-accessible refuelling stations. Complete coverage of Britain will require an estimated 1,000 H2 filling stations but without these, few car and light commercial vehicle buyers would be willing to purchase HFEVs.
To overcome this conundrum, governments could consider streamlining regulations and creating a secure long-term business case to entice private investors.
Emergency services
For road authorities and emergency services, the biggest challenge EVs pose is probably a fire or crash although when a BPV’s airbags are triggered, the main battery is automatically isolated, immobilising the vehicle. Early indications are that EV fires are less frequent than with ICE and hybrid vehicles but when they do catch fire, they make headlines.
If they do catch fire, EVs (HFEVs have a small battery too) can cause big problems as lithium burns at around 2,000°C, gives off toxic fumes and can reignite over a period of many hours. After attending a BPC fire in Austin, Texas, the local fire department said it took 40 times the amount of water to put out the blaze than with an equivalent petrol vehicle. These factors will have a significant impact on the equipment carried by emergency vehicles.
Colin Glenholm, MD of Autotech Training which trains staff from emergency services, advocates the use of fire blankets which are pulled over the burning EVs to starve the fire of oxygen, reduce the temperature and so extinguish the flames. Either way, the disruption (and possible road/infrastructure damage) caused by a BPV fire would be significant.
Also concerning is that H2 is explosive in concentrations between 4% and 75% although it disperses far more readily than, for instance, petrol. HFEVs also have numerous safety systems to detect and stop leaks including fuel tanks that can withstand being shot at or being caught in a fire (using a controlled release of H2 to relieve over-pressurisation).
In its publication A Risk Assessment of Hydrogen Fuel Cell Electric Vehicles in Tunnels, Sandia National Laboratories in Albuquerque concluded there is a 98.1 to 99.9% probability that there would be no additional hazard from the hydrogen fuel beyond the existing hazards in a vehicle crash.
Nonetheless, the potential for a slow accumulation of gas in enclosed parking lots and underground garages must be considered by road, parking and building standards authorities, ferry operators and others. Consideration of regulation on acceptable parking locations, ventilation requirements and explosion-proof electrics may be pertinent.
In view of the intense heat EV battery fires create, authorities may also want to reconsider the desirability of enclosed parking areas and recharging points below apartment blocks, offices and shopping malls.
Such considerations are leading to different treatment of BPCs and HFEVs. One example is Eurotunnel which allows BPCs on its LeShuttle service but not HFEVs.
There is no ‘neutral’ on an EV and they all have regenerative braking; therefore, they cannot be towed with their driven wheels on the ground which creates challenges for breakdown and crash-recovery services. UK recovery specialist, the AA, has developed a freewheeling hub wheel that replaces the driven wheels when towing a stranded EV or 4WD or when the wheel/brakes have seized. It has also added mobile battery recharging units to its roadside assistance vehicles to get stranded EVs moving again.
Commercial vehicles
BPVs’ weight penalty can be problematic for commercial vehicles although local authorities can lead the way. For example, electric truck manufacturer Electra builds refuse collection vehicles on OEM chassis and fits battery packs sized to last the full shift. According to its chairman Sid Sadique, the trucks’ payloads are between 250kg and 500kg less than their diesel-powered counterparts although domestic waste will fill the bodies before the trucks become overweight. And while the upfront cost is higher, Sadique says whole-life costs are on a par with, or lower than, the diesel-powered counterpart - and with zero tailpipe emissions.
Many cities have introduced hydrogen-powered buses and many HFEV truck trials are under way, including in Switzerland where 23 companies are using 47 Hyundai trucks that have collectively clocked up five million kilometres (3.1 million miles). A UK supermarket’s trial of Electra’s 19t refrigerated HFEV truck found it saved 314kg of CO2 each day on a 335km (208 miles) round trip.
With battery-powered vans the problems are more acute. Ford’s battery-powered 3,500kg L2H2 Transit van loses 370kg of payload allowance against its diesel-powered equivalent and its load floor is at least 100mm higher. Allowing battery-powered vans slightly higher axle/gross weights without requiring a higher class of driving licence or attracting higher taxes would help address this disadvantage.
In poor air quality areas, authorities could set early deadlines for professional fleets (HGVs, buses, taxis, private hire vehicles…) to transition to zero tailpipe emission vehicles. This would incentivise sales, lower emissions and improve air quality without rushing to penalise private households where an EV is either unsuitable or unaffordable.
Small changes to legislation, grants and tax breaks could greatly ease the decarbonisation of road transport by incentivising buyers and unlocking private equity to develop and build zero tailpipe emission vehicles and create refuelling infrastructures.
Energy supply
Decarbonising transport will have a colossal impact on electricity production and distribution systems but the technology could also help address renewable energy’s biggest problems: unpredictable and mismatched generation and energy storage.
Most BPVs will be charged at home overnight when demand on the grid is generally lower but it often peaks between 5pm and 9pm, leading many countries to require domestic chargers to be internet-connected, ‘smart’ and pre-programmed to avoid charging in peak periods. These devices also stagger start times and ‘smooth’ the end of charging to prevent sudden rises and falls in electricity demand (especially with timed charging).
Utilising a BPC’s battery to cover power outages could be useful but would require both technology and regulatory changes far beyond the jurisdiction of DoTs.
Although electrolysing water to produce hydrogen is energy-intensive and will increase overall electricity demand, production can be ramped up in periods of low electricity demand or when excess renewable electricity is available, and stored until needed. Then, in periods of high demand, production can be reduced or stopped and the stored H2 can even be turned back into electricity to cover grid shortfalls or outages.
Prefabricated ‘plug and play’ electrolysers for H2 production could suit fleets and service stations: for example, the ones ITM Power builds into ISO containers use 2MW of electricity to generate up to 36kg of hydrogen per hour. That equates to 1.2MW of stored H2 energy per hour which would power Hyundai’s Nexo for 3,600km (2,240 miles), a performance roughly equivalent to a frugal ICE.
BPV drivetrains are typically 85-90% efficient and after allowing 15% losses during battery charging, all BPCs would exceed 3,600km on 2MW of (dispensed) energy even in cold weather and most would do twice that distance in warmer weather (above 12°C).
Trials of H2-powered jet planes are also under way as are feasibility studies of converting all domestic (and commercial) gas appliances to run on low-pressure hydrogen supplied through existing gas distribution network(s). The ongoing war in Ukraine has brutally revealed the vulnerability of centralised energy production and many countries have suffered the downside of relying on imported energy.
It is incumbent on the transport sector to lead the way in a global shift away from fossil fuels but all sectors of government, national and local, need to be involved because this cannot be achieved from inside silos.
What about fuel duty?
Many governments need to identify methods of collecting duty from EV users that are as easy to administer and proportional to road usage as taxing petrol and diesel.
Imposing duty on the electricity dispensed via EV charge points may appeal but would tax any renewable energy produced by systems installed by individuals and businesses, making it a deterrent to investing in renewable energy and EVs. Furthermore, BPC owners could avoid the duty by using slower (3kW) chargers that plug into the domestic socket and in doing so would also bypass the peak-demand management.
The H2 for fuel cell vehicles could be taxed at the point of delivery as with petrol and diesel although service stations, large transport companies and perhaps farmers could become producers by installing H2 generation plants – which would increase complexity.
Alternatively, a combination of road fund licence and mileage tax (by vehicle type, weight etc.) would be relatively simple and proportional to road use, particularly as some cities are considering introducing their own ‘smart’ road-user charging system for mileage clocked up within their boundary which would compound the complexity of a national system and confuse motorists. A mileage tax system would also cope with any new propulsion variant.
Clean, yes - but green?
All transport comes with environmental costs: burning a litre of petrol creates almost 2.4kg of CO2 (diesel exceeds 2.6kg) while the ‘tailpipe’ equivalent for EVs is zero and reducing CO2 emissions is key to combatting climate change.
However, the way EV ‘fuel’ is created is a far greater environmental problem that can be fixed. If BPVs’ batteries are charged with electricity generated by burning fossil fuels, then the emissions simply move from the road to the power station whereas when using renewable sources, the environmental benefit is substantial.
HFEVs pose a similar problem as currently more than 90% of the world’s H2 is ‘grey hydrogen’ made from fossil fuel in a greenhouse-gas-releasing process that cancels out any wider environmental benefit. Yet more environmentally damaging is using fossil-fuel-generated electricity to produce H2 via electrolysis, while utilising renewable energy produces carbon-free ‘green hydrogen’.
The US EPA has examined the evidence and concluded that: ‘Electric vehicles typically have a smaller carbon footprint than gasoline cars, even when accounting for the electricity used for charging’, while the EU’s Hydrogen Roadmap says building HFEVs requires ‘significantly less raw materials than batteries and combustion engines’.
It is a fact that lithium mining is more environmentally destructive than oil extraction - however, lithium batteries will power BPCs for about 10 years. They can then be used in less demanding (often domestic) applications by the likes of Eaton’s xStorage Compact which can reuse Nissan Leaf batteries. Much research is also under way to devise efficient lithium battery recycling methods that do not create toxic waste.